Russian robot-telescope MASTER in Argentina detected Space Chernobyl 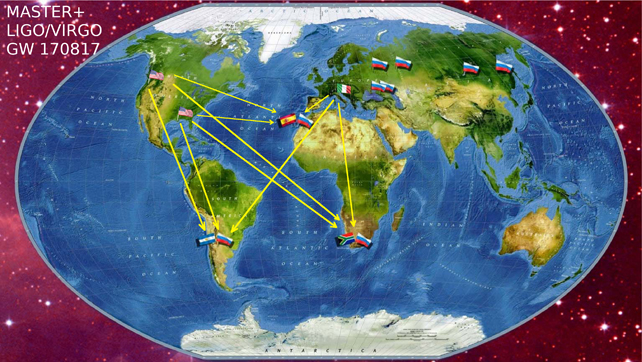

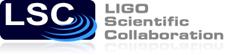 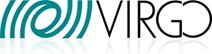
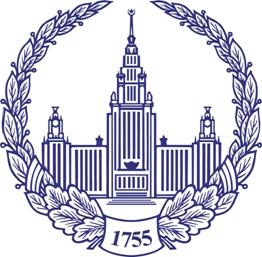
Press release
MASTER GLOBAL ROBOTIC TELESCOPE NET OF MOSCOW STATE UNIVERSITY
The first detection of the collision of neutron stars and optical
KIONOVA explosion
August 17, 2017
(Birth of gravitational-wave
astronomy)
Neutron Stars
Merging Discovery
The gravitational-wave discovery of neutron-star merger
on August 17, 2017, GW170817/GRB170817a, accompanied by a gamma-ray burst and optical
"kilonova" is a triumph of our views about the evolution of matter in
the Universe. Optical flash was discovered independently by several telescopes
including instruments of MASTER global robotic NET. The discovery of a
neutron-star merger at a distance of 40 Mpc is completely consistent with the first
population-synthesis computations made 30 years ago at Moscow State University
with the Scenario Machine.
We can now conclude that a new science –
gravitational-wave astronomy – was born.
What happed on August 17, 2017?
Three gravitational-wave antennas located in the USA (Louisiana,
Hanford) and Italy (Pisa) detected practically simultaneously the collision of
two neutron stars at a distance of 40 Megaparsecs. The coordinate error was
about one hundred degrees. However, 2 seconds after that event the
omnidirectional antenna of NASA’s Fermi gamma-ray observatory detected a short
gamma-ray burst,. Which was later confirmed by European INTEGRAL and AGILE
gamma-ray observatories.
Further refining and correcting of the possible
direction in the sky reduced the error box size to about 100 square degrees. After
half a day several ground-based optical telescopes including one of the eight Russian
robotic telescopes of the MASTER Global Robotic NET of Moscow State University
located in Ands 2500 m about the sea level (Argentina, Observatory of National
University of San Juan) detected an optical flash in the NGC 4993 galaxy. Why
so fast?
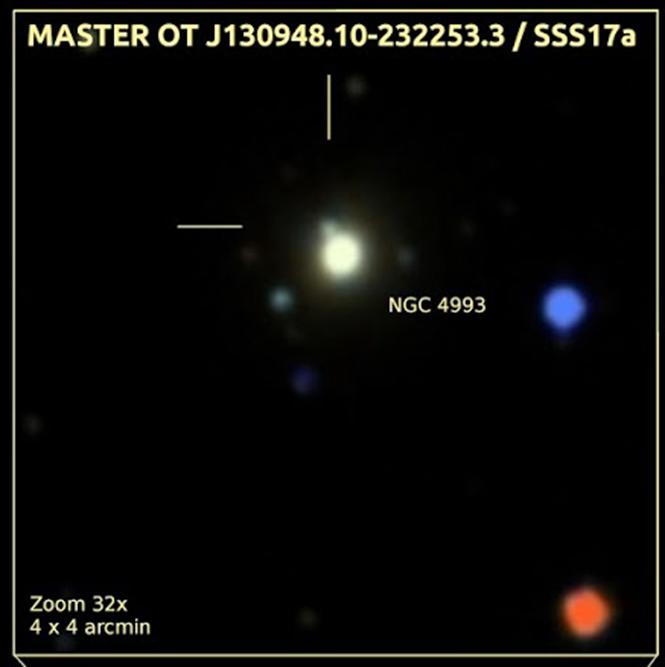
Fig.1.
Localization of the merger of the binary neutron star. The two unconnected
light-green areas show the initial error field based on the data from LIGO gravitational-wave
antennas. The dark-blue oval area shows the error field of the gamma-ray burst GRB170817A
determined by Fermi gamma-ray observatory. The semitransparent strip shows the
error field obtained by the international team of researchers from Ioffe
Physical-Technical Institute (St.-Petersburg, Russia) and University of
California (Berkeley, USA) based on the data from Fermi and INTEGRAL gamma-ray
observatories. The dark-green area shows the final localization of the event
obtained with all the above data supplemented with the data from VIRGO
gravitational-wave antenna. The right-hand part of the figure shows the optical
images of the galaxy and kilonova obtained by MASTER Global Robotic NET of
cosmic monitoring.

Fig.2. The telescopes of MASTER Global Robotic NET obtained
the first independent images of the NGC 4993 galaxy
after the reported detection of gravitational-wave signal GW170817/ G298048 by three LIOGO-Virgo
antennas (Abbot et al., 2017). On these images the optical transient MASTER OT J130948.10-232253//SSS17 was later detected, which represents a new type of astronomical
explosions - Kilonova. The Russian flags indicate the locations of the
telescopes of MASTER Global Robotic NET ( http://observ.pereplet.ru ). The two
American and one Italian flags indicate the locations of LIGO/VIRGO
gravitational-wave antennas.
For example, the telescopes of the 20th century could
not detect optical emission of the most powerful explosions in the Universe –
gamma-ray bursts – for 30 years. This is not surprising given that it would
take several weeks to observe the entire error ~100 square-degrees large error
box of GW 170817 not to say about processing the acquired images.
How could a similar task be achieved with several
hours? The answer is simple – this became possible because of the revolution in
the speed of search telescopes that occurred in the past 20 years. The
capabilities of such telescopes increased hundredfold! Now the fastest robotic
telescopes are capable to observe the entire sly in a week. In the 20th
century such a task would take several decades to achieve. And one has not only
to obtain the images, but also detect all new
objects that appear on them!
Hence the event of August 17, 2017 was a result of a combination
of two technological revolutions: physicists learned how to determine distances
with thousand times better precision compared to 20th century and astronomers
made robotic telescopes capable of observing sky fields and detect new objects
at a thousand times higher speed.
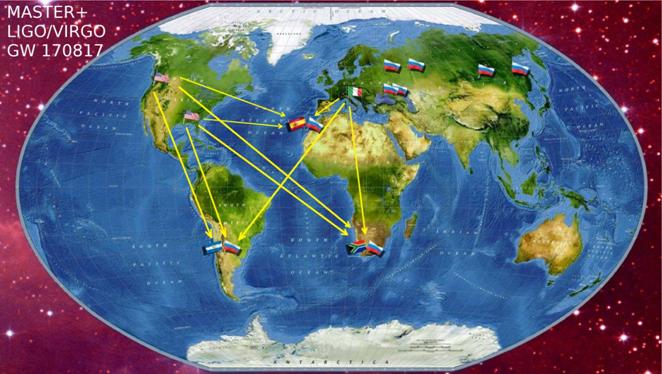
Fig
3. Chronology of the GW170817/GRB170817/SSS17/MASTER OTJ130948.10-232253.3 discovery
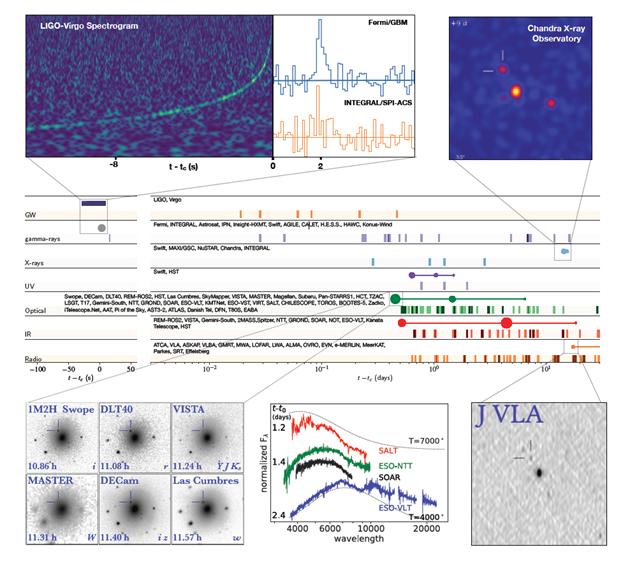
Fig.4. Robotic telescope of MASTER NET at Observatorio
Nacional Felix Aguilar (National University of San Juan, Argentina), June,
2016. Right to left: Evgeny Grabovskoi, Igor Gorbunov, Prof. Vladimir Lipunov (head
of the project), Ricardo Podesta – Director of OAFA, Dr. Carlo Safe (Instituto
de Ciencias Astronómicas, de la Tierra y del Espacio, San Juan) and Federico
Podesta (OAFA). MASTER robotic telescope was installed with the support from
Sergei Mikhailovich Bodrov (AO «Optika»).
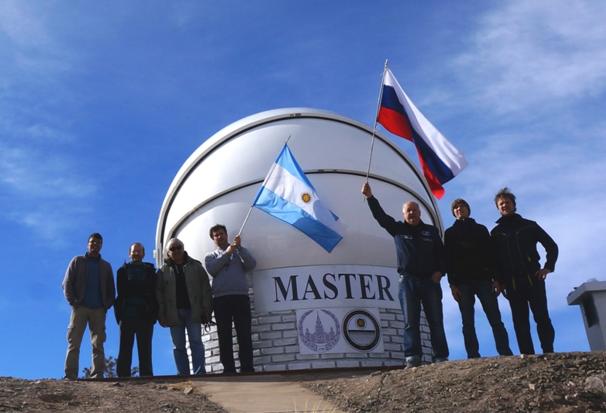
Fig.5. Composite image of the explosion of August 17, 2017 in
the NGC 4993 galaxy obtained on MASTER telescopes in South African and
Argentina.
Neutron stars represent the first class of astronomical
objects that were predicted theoretically and confirmed by observations. In 1932
the Soviet physicist Lev Davydovich Landau suggested that there must exist
giant atomic nuclei with masses greater than the solar mass and with sizes of
about 10 km. This happed even before the discovery of the neutron by James
Chadwick. Two years later, in 1934, the American astrophysicists Walter Baade
and Fritz Zwicky called these giant nuclei neutron stars and suggested that these
stars are born as a result of catastrophic collapse (gravitational contraction),
which, in turn, is accompanied by a supernova explosion. Baade and Zwicky pointed
out the Crab nebula, which formed as a result of a supernova explosion observed
by Chinese astronomers in 1054. And it was in the Crab nebula that the youngest radio pulsar – a rapidly rotating
neutron star – was discovered after 35 years.
In
1966 the Soviet scientists Yakov
Borisovich Zel’dovich and Igor Dmitrievich Novikov (1966) found the physical
process, which could make these very small objects (compared to stars) with
radii of about 10 km bright sources of electromagnetic radiation. This mechanism
– infall of surrounding matter onto the neutron star – was suggested by Iosif
Samuilovich Shklovsky (1967) to explain the nature of the brightest x-ray
source Sco X-1. Almost at the same time Nikolai Semenovich Kardashev (1964) and
the Italian astrophysicist Franco Pancini (1967) found another source of energy of a magnetized neutron star
– the rotational energy of the star accumulated during its collapse. Thus neutron
stars born at the point of the pen became a scientific hypothesis, which was
directly confirmed after the discovery of radio pulsars by the British radio
astronomer Antony Hewish, (1974; Nobel Prize 1971) and x-ray pulsars (Riccardo
Giacconi, Nobel Prize 2002).
After the
discovery of a binary radio pulsar by the Australian radio astronomers Alan
Hulse and Joseph Taylor (Nobel Prize 1993) it became clear that neutron-star
collisions should occur in the Universe because the merger time of this binary
was found to be smaller than its age (Brumberg, Novikov, Shakura, et al.,
1975).
The process
of collision of two neutron star - these
supermassive atomic nuclei- resembles the collision of elementary particles in
colliders. However, the energy released in this peculiar cosmic collider is
incomparably greater. In fact, collisions of neutron stars long with collisions
of black holes, which were discovered two years ago (this discovery was recently awarded the Nobel
Prize in physics for 2017), is the most powerful process in the Universe, which
is accompanied by a gravitational-wave pulse. That is why Kip Thorne – the
chief ideologist of the project -
started promoting the idea of LIGO gravitational-wave detector back in 1980ies.

Photo 1. The 2017 Nobel
Prize Winners physics. The Nobel Prize was awarded for the discovery of
gravitational waves in 2015.
However, a
question immediately arose: how often do such processes occur in the Universe? Or,
putting this in terms of particle physics, one had to compute the probability
of collisions of relativistic stars in the Universe – the cross-section of the
most powerful cosmic reactions.
The first
attempts of estimating the neutron-star merger rate in our Galaxy based on general
views about the evolution of binary systems up to the formation of relativistic
stars proved to be rather approximate: 10-4
- 10-6 mergers/year. Why? Because the
merging rate is equal to the product of many difficult-to-estimate probability factors
like those appearing in Drake’s formula for the number of habitable planets in
our Galaxy.
Fortunately, in
early 1980-ies young Soviet astrophysicists Viktor Kornilov and Vladimir
Lipunov, who had just finished their post-graduates studies at Sternberg
Astronomical Institute of Moscow State University, invented a new theoretical
method for the study of the Universe – the
Scenario Machine. The main idea of the
Scenario Machine was to develop a computer model of our Galaxy and then of the
entire Universe. In such Simulated Universe simulated binaries were born continuously,
which lived in accordance with our theoretical views about the evolution of
binary systems. Of course, these underlying concepts might not be very accurate,
but even now no accurate model is available. The initial parameters of binary
systems were sampled randomly using what mathematicians call the Monte-Carlo
method. The young astrophysicists used various scenarios of the evolution of
binary stars trying to chose such evolution parameters that would best explain
the observed stages of the evolution
of binary systems. I.e., the simulated Universe must at a certain stage contain
objects like Cyg X-1 – a black hole with a blue supergiant.
The Scenario Machine for computing the
scenarios of the evolution of binary stars was a decade ahead of Western
research in this field. This happened because of the unprecedented concentration
of astrophysical astrophysical thought around one of the creators of Soviet
nuclear weapons – Academician and – dreadful to think – triple Hero of
Socialist Labor - Yakov Borisovich
Zel’dovich. Even the future ideologue of the LIGO project and now a Nobel Prize
Winner - Kip Stepanovich Thorne (this is
how he was called in the team of Yakov Borisovich Zel’dovich), must have gained
this idea at seminars held by Yakov Zel’dovich (now Zel’dovich All-Moscow
Seminar of Astrophysicists), whom he visited regularly since early 1960-ies. Indeed,
the idea of gravitational-wave antenna was suggested by Soviet physicists
Pustovoit and Gertsenshtein in early 1960-ies. It was at Zel’dovich’s seminar
that Kip Thorne met Vladimir Borisovich Braginsky – the head of the team of researchers
of the Faculty of Physics of Moscow State University, which made an
indispensable contribution to the success of the gravitational-wave experiment. (See http://www.pereplet.ru/lipunov/372.html#372 ; http://www.pereplet.ru/lipunov/368.html#368
about the contribution of Soviet researchers to the first detection of
gravitational waves on September 14, 2015).
During one of his visits Kip Thorne learned
about the new tool developed by Russian researchers and asked them to compute
the probability of collisions of neutron stars.
This is how the probability of collision of
neutron stars in our Milky-Way Galaxy came to be computed for the first time. It
turned out that such event should occur once in 10 000 years in our stellar
home. This was found out in 1987, when the former Zel’dovich’s post-graduate student developed the new
Scenario Machine, now together with his graduate and post-graduate students (V.M.Lipunov,
V.M.Postnov, and M.E.Prokhorov, 1987). It was now very easy to tell Kip
Stepanovich what should be the “range” of his gravitational-wave antenna to get
Nobel Prize for the discovery of gravitational waves. The antenna should reach
such a distance that the sphere of the corresponding radius would contain 10
000 galaxies. In this case the antenna should detect gravitational waves at
least once a year. The radius of this sphere turned out to be equal to 20 Megaparsecs
or 60 million light-years. However, it would be better to detect at least
several events each year and hence the interferometer horizon should be
increased at least to 40 Megaparsecs to record several events each year.
Somewhat
later the American astrophysicist Hills
(1990) and Soviet astrophysicists Tutukov and Yungel’son (1993) obtained
similar estimates. In 1999 Hans Bethe – a Nobel Prize Winner (1967) and one of
the discoverers of solar thermonuclear energy – made the last attempt to
estimate the merger rate analytically.
We
show in Fig. 3 a very expressive plot – predicted neutron-star merger rate as a
function of the antenna sensitivity horizon (Lipunov et al., 2017a). The vertical
gray stripe indicates the distance to the NGC 4993 galaxy with the present-day
error interval. The dashed lines show the prediction of the Scenario Machine. It
is evident from the figure that the discovery of colliding neutron stars at a
distance of 40 Megaparsecs (120 million light-years) agrees excellently with
the results of the computations made in 1987! Note that a number of researchers
in other countries reported 10-100 times lower merger rate estimates.
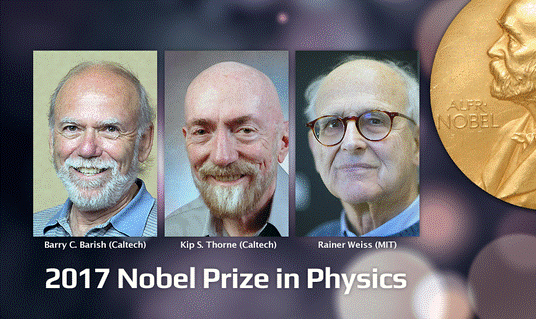
Fig.
6. Neutron-star merger rate as a function of the sensitivity horizon (see Fig.6.
in Lipunov & Pruzghinskaya, 2014) with the GW170817 event superimposed. The
dashed lines show the results of the computations made with the Scenario
Machine. The red asterisk shows the lower limit of 2012, which was consistent with
our predictions. The vertical gray strip shows the estimated distance to the NGC4993
galaxy with real error limits: 41+/- 5.8 Mpc. The horizontal gray strip shows the prediction
if the Scenario Machine – several events/year! Excellent agreement given that
gravitational-wave antennas had been operating for about 1/3 year (Abbot et al.,
2017)!
Thus on August 17, 2017 at 12:41:04.44 UT LIGO/VIRGO gravitational-wave observatories (USA-Italy) detected the collision of two neutron stars at a distance of 120 million light-years from the Earth. Two seconds later NASA’s Fermi and ESA’s Integral gamma-ray observatories detected a short pulse of gamma-ray radiation – a gamma-ray burst. After about 10 hours wide-field cameras of MASTER robotic telescope in Argentina acquired an image of the galaxy where the catastrophe occurred, and somewhat later MASTER telescopes and several American telescopes in neighboring Chile detected a new 17.5-magnitude object.
It is
remarkable that the optical object discovered 12 hours after the merger in the NGC
4993 galaxy did not resemble any of the
supernovas studied so far neither in behavior nor in brightness or spectrum.
The soon acquired optical spectra of the object confirmed that the envelope of
the kilonova expands at a velocity of 100 000 km/s, i.e., one third of the
speed of light, which corresponds to the escape velocity at the surface of
neutron stars.
Thus on Agust
17, 2017 the astronomers and physicists observed almost simultaneously the merger
if two neutron stars and its consequences in the NGC 4993
galaxy not only in the gravitational-wave channel, but also in several ranges
of electromagnetic radiation from gamma- and x-ray radiation to ultraviolet,
optical and infrared.
Despite the unique nature of this event, the variety of
experimental data already allows us to make important theoretical conclusions
about the origin of binary neutron stars, their mergers, and accompanying
bursts of electromagnetic radiation.
Below I will tell you how the gravitational-wave,
gamma-ray, and optical bursts were detected, how this event fits our views, and
what new knowledge did this discovery bring.
There are several reasons why neutron-star merger was expected to be accompanied by electromagnetic radiation. Soviet astrophysicists Sergei Ivanovich Blinnikov and Igor Dnitrievich Novikov were the first to point out this connection in their paper published in 1984. In 1998 Professor Bogdan Paczynski (Princeton University) together with his post-graduate student found that after the collision of neutron star part of the nuclear matter may be ejected back into space. Nucleons – protons and neutrons – should then almost immediately begin to combine into heavy radioactive atoms of Mendeleev’s periodic table. Their decay should result in an optical burst several hours after the explosion. This burst will be weaker than the supernova explosion, but it still will be thousand times brighter than novas. That is why these explosions , which were then hypothetical, were called "Kilonovas". And it is this very sort of event that was first confidently discovered on August 17, 2017!
Beginning with the first gravitational-wave event recorded by LIGO interferometers (Abbot et al., 2016 , Lipuniv. Uspekhi Fiz. Nauk 2016), the MASTER Global Robotic Telescope NET has been taking active part in the search for optical emission of all detected LVC events (Lipunov et al., наблюдения GW 150914, 2016), thereby making the greatest contribution to the study of GW 150914 in the optical (Abbott 2016a,b). On August 17, 2017 at 12:41:06.47 UT von Kienlin et al, 2017 reported that the Gamma Burst Monitor (GBM) installed onboard Fermi observatory recorded a short (2-sec long) pulse – a gamma-ray burst, - which occurred 2 seconds after the detection of the gravitational-wave event.
These
discoveries and the subsequent observations showed quite conclusively that on
August 17, 2017 the astronomers observed for the first time a collision of two
neutron stars and its consequences in the NGC 4993 not just in the gravitational-wave channel, but also
in several ranges of electromagnetic radiation from gamma- and x-ray radiation
to ultraviolet, optical and infrared.
Despite the unique nature of this event, the variety
of experimental data already allows us to make important theoretical
conclusions about the origin of binary neutron stars, their mergers, and
accompanying burst of electromagnetic radiation (Lipunov et al., 2017b).
Discovery.
The first information about possible coordinates of
the neutron-star merger came from LIGO/VIRGO gravitational-wave antennas and
the huge error box of Fermi gamma-ray observatory (see Fig. 2.) at about noon
Unuiversal Time. At that time of all MASTER sites it was night only at Blagoveshchensk,
but MASTER-Amur telescope was not operating because of weather conditions. Hours
passed and the night shadow crossed Russia, but, as ill luck would have it, bad
weather everywhere made it impossible to conduct observations. Only at 17:06:47
UT, i.e., 4.42 hours after the detection of the gravitational wave, the Sun was
down in South Africa and our MASTER-SAAO telescope (South Africa Astronomical Observatory)
automatically stared inspecting the huge (more than one thousand square
degrees) error field in the sky. It was found out later that the galaxy where the
event occurred went under the horizon rather soon before the telescope covered
the corresponding area. At that time night fell on Canary Islands, where the
Russian MASTER-IAC (IAC = Instituto Astrofisica di Canarias) is located.
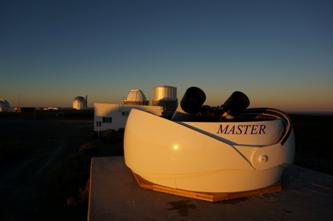
Photo 1. MASTER-SAAO (South
Africa) and MASTER-IAC (Canary Islands, Spain) were the first telescope to
start searching for the event location. However, at the time the error box was
too large...
At 20 hours 29 minutes 26 seconds UT (7.80 hours after the trigger) the telescope
on Canary Islands started searching for
the event location. At that time we already new the final (refined) and small (~100
square degrees) banana-shaped error box in the Southern Hemisphere, – but it
was already under the horizon.
It has to be said that starting from 15 hours UT the MASTER
team was floating down the Moskva river on a boat enjoying the beautiful Moscow
evening of August 17, 2017. I would stretch the truth should I not mention that
it was the fellowship banquet dedicated to the "Exploding Universe in
Robots’ Eyes" meeting (http://master.sai.msu.ru/ru/master2017/ ) .
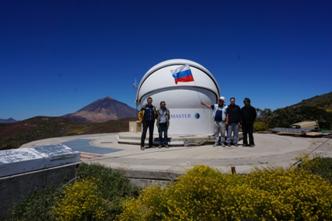
Photo 2. Participants of the
international meeting "Exploding Universe in Robots’ Eyes" dedicated
to the 15-year anniversary of the MASTER project ( http://master.sai.msu.ru/ru/master2017/
).
It was at on this boat, while drifting by the Bell
Tower of Ivan the Great, that Dmitry Svinkin – a participant of the meeting
representing the glorious Ioffe Physical-Technical Institute whispered me
reading the LVC GCN circular reporting the discovery of the neutron-star merger!
When we all returned to our homes Dmitry Svinkin used
the triangulation method to refine the supposed coordinates of the catastrophe
and the final error box, reducing it to less than 100 square degrees. We fell asleep
without even knowing that our MASTER-ОАFA robotic telescope had already started observing it.
Фото Photo 3.
June 2016. Construction of the MASTER telescope in Argentina is almost
finished. Observatorio Astronomico Felix Aguilar of National University of San
Juan.
The MASTER telescope in Argentina took its first image at 22:54:18 UT, i.e., 10.22 hours after the collision and, as it turned out later, MASTER teklescopes missed the NGC 4993 galaxy. However, our wide-field cameras with a 380 sq. degrees large field of view covered almost the entire error box together with the locus of the neutron-star collision. 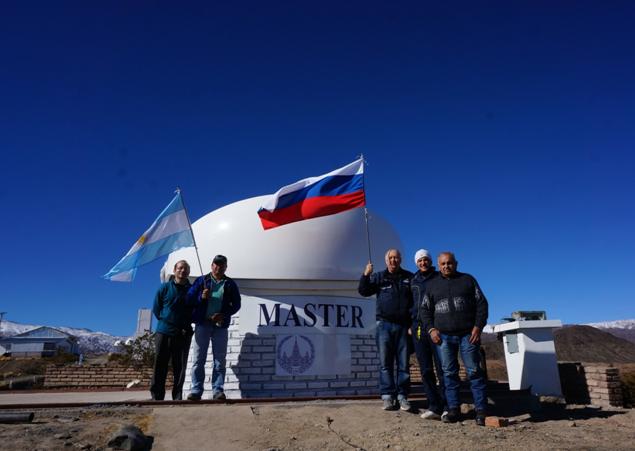 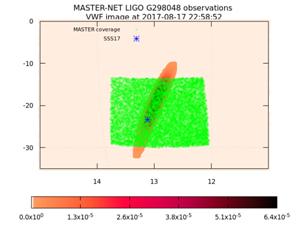
Fig.А.The first image of the NGC 4993 taken after the grand event of the collision of two neutron stars. The strokes in the right-hand image indicate the first post-event image of the NGC 4993. An analysis of the image yielded an upper limit of ~15.2m for the brightness of the flash at that time. Exactly one hour later, 6 seconds before the end of August 17, 2017 UT, MASTER telescopes came across the galaxy where the historical event had occurred. It was a good birthday present for MASTER. 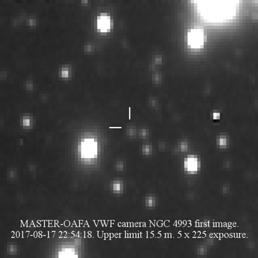
Fig. B. Map of the Argentinian MASTER image of the final error box. It was 6 seconds before midnight UT when MASTER came across what remained of the merger of two neutron stars. And early in the morning, when we got up, we read the telegram from Swope telescope, which took the image of the kilonova in the NGC 4993 galaxy located at the very distance of 40 Mpc from the Earth. During the night our telescope independently! Detected the new object in the NGC 4993 galaxy. 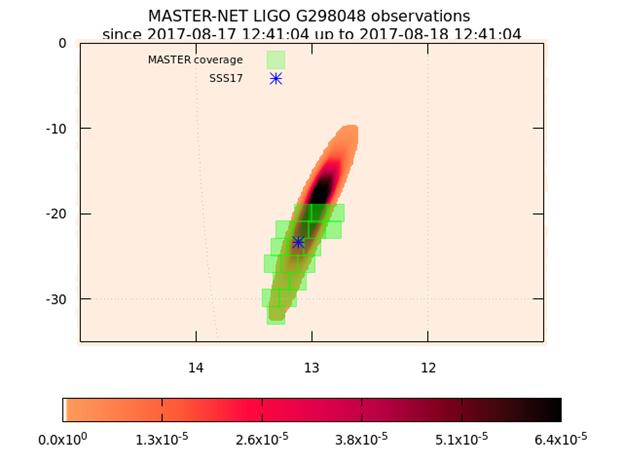
Fig. 5. The first image of the kilonova obtained
wityh MASTER-OAFA robotic telescope in Argentina at 2017-08-17 23:59:54 Universal
Time. The left image was taken on August
17, and the right-hand image shows the reference frame taken before several
month. The middle image shows the difference frame. As is evident from the
difference frame, a new object – a kilonova – has appeared in the galaxy.
On the next night we were already taking images of the likely sky areas. - When they came across this place in our manuscript the editors of the Astrophysical Journal Letters asked us - Why, it could be just an ordinary supernova? We added a couple of phrases to our paper. The point is that at daytime on August 18 a telegram came reporting a very simple estimate of the expansion velocity of the shining ejected shell, and this velocity turned out to be equal to ~ 1/3 of the speed of light. It is about 10 times higher than the expansion velocity of the fastest supernova shell. Furthermore, a number of telescopes managed to acquire the spectra of the object and it turned out that the astronomers have never seen anything like that neither in supernova or nova spectra. It was a real Chernobyl! Hundreds of lines of superheavy chemical elements. It became absolutely clear that the object in NGC 4993 was the very kilonova described by Bogdan Paczynski, which had to appear after the neutron-star merger. And during several nights that followed we, like dozens of telescopes worldwide, simply took images of the now famous object. However, the kilonova faded in just three days, and we could not see it any more in our 40-cm MASTER telescope – it was the smallest instrument that could stand on a par with giant telescopes (including the 4-meter VISTA telescope). And Swope telescope was the first to report the discovery. It has an interesting history. It is a 40-inch (1-meter) telescope installed in Chilean mountains in 1971 and upgraded to perform a sky survey aimed at the discovery of supernovas in the Southern sky. It was named after the astronomer Henrietta Swope – a teammate of the great astronomer Walter Baade, who funded the project. This is how the greatest discovery in astronomy and physics was made. October 16, 2017. Moscow, M.V.Lomonosov Moscow State University, Sternberg Astronomical Institute, 16-00
Popular science publications
of V.M.Lipunov on the subject of the paper.
1) V.M.Lipunov, V
mire dvoinykh zvezd (In the world of binary stars), Nauka: Moscow, 1986 (Bibluioteka
Kvant). New edition, in Russian
2) V.M.Lipunov, Iskusstvennaya
Vselennaya (Similated Universe), Sorosovskii obrazovatel’nyi zhurnal, 1998, N6,
82-89, , in Russian
http://nuclphys.sinp.msu.ru/mirrors/1998_6b.pdf
3) V.M.Lipunov, Gravitatsionno-volnovoe nebo (Gravitational-wave
sky). Sorosovskii obrazovatel’nyi zhurnal, 2000,N , 77-83, , in Russian
http://www.pereplet.ru/lipunov/0004_077.pdf
4) V.M.Lipunov,
"Voennaya taina astrofiziki " (Astrophysic’s top secret), Sorosovskii
obrazovatel’nyi zhurnal, 1998, N5, c.84-92, , in Russian.
http://www.pereplet.ru/nauka/Soros/pdf/9805_083.pdf
Publications of the MASTER team and papers
accepted by journals dedicated to the GW170817 event.
The papers will be
published on October 16, 2017.
1.Abott et al.,
2017, MULTI-MESSENGER OBSERVATIONS OF A BINARY NEUTRON
STAR MERGER, ApJlett,
2. Lipunov et al.,
2017 , MASTER optical detection of the first LIGO/Virgo neutron stars merging
GW170817, ApJlett. https://doi.org/10.3847/2041-8213/aa92c0
3. B. Abbott1 et al., A
gravitational-wave standard siren measurement of the Hubble constant, Nature, accepted
4. Lipunov, V.M.
et al., Discovery of the neutron
stars merger GW170817/GRB170817a and Binary Stellar Evolution, New Astronomy Review,
5. Buckley, D.
et al., A comparison between SALT/SAAO observations and kilonova models for AT
2017gfo: the first electromagnetic counterpart of a gravitational wave
transient − GW170817, MNRAS 000, 1–4 (2017)
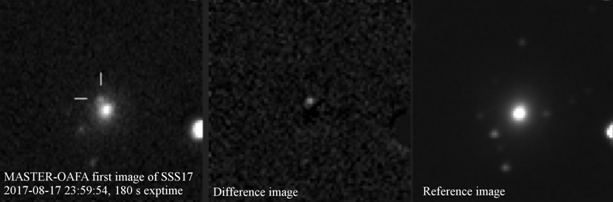
Vladimir Lipunov<.I>
|